BMI (hardware) review
Introduction
You may have heard of brain-machine interfaces (or brain-computer interfaces) thanks to the very public announcements made by companies such as Neuralink. Very much in accordance with Musk’s strategy of publicly decrying outlandish future achievements to realize on an impossible timescale, he has made some fairly awesome claims for Neuralink’s future. These include, for example, ending the need for verbal communication within 5-10 years. Although these claims will probably take longer to come to fruition, if they ever come at all, they highlight the exciting potential of this truly transformative technology.
The aim of this post is to quickly review what has already been achieved in the field of BMIs, separate the grounded from the fantastic and provide a general scope of what is being achieved in this field.
What are they?
My old friend Wikipedia tells me a BMI is a “direct communication pathway between an enhanced or wired brain and an external device.” It seems this definition is intentionally quite broad. Indeed, a direct communication pathway can mean many things. The word direct in itself is subject to interpretation, how direct is a non-invasive interface like the one developped by (formerly known as) CTRL-Labs and now part of Facebook Reality Labs which is non-invasive and worn on the arm? Don’t get me wrong, this tech is frickin’ cool, but it just goes to show that ‘direct’ can be loosened a little. Furthermore, a communication pathway can allow for many different communication protocols, can be one-way or both and if we consider neuroimaging techniques such as fMRI scanners to be BCIs, the so-called pathway can be highly non-portable and burdensome. I’ll focus here on BCI technologies using electrical activity generated by the brain or the muscular system rather than other potential BCI modalities using magnetic fields, absorption spectra of near-infrared light, or blood flow. I will, therefore, willingly restrict my analysis to technologies fitting the definition given by nature: “A BMI is a device that translates neuronal information into commands capable of controlling external software or hardware such as a computer or robotic arm.” Going by said definition, the BMI should be able to (i) record neuronal information and (ii) translate it. This naturally distinguishes the ‘hardware’ i.e the recording technology, from the ‘software’ i.e the processing and decoding algorithms making sense of the captured signals. The software side of things will (if I ever get to it) be the subject of a later post as this is the fascinating algorithmic aspect of BMIs.
From my limited knowledge on the topic, technology capable of doing what is mentioned above usually involves tracking electrical activity generated by ensembles of cortical neurons or the musculo-nervous system. These can be separated into two broad categories. The first is invasive BCIs, that is they require implantation of some part of the device directly into the brain. Invasive technologies could further be broken down between single site recordings and multi-site recordings if we really want to push the nomenclature further. The second overarching type is, you guessed it, non-invasive BCIs. For those of you who feel a little uneasy about having a foreign body implanted directly into your brain tissue, you may be happy that more than a few researchers and companies are working on the second approach. Personally, I don’t really see the problem with the former, provided the safety, efficacy and usefulness have all been tried and tested.
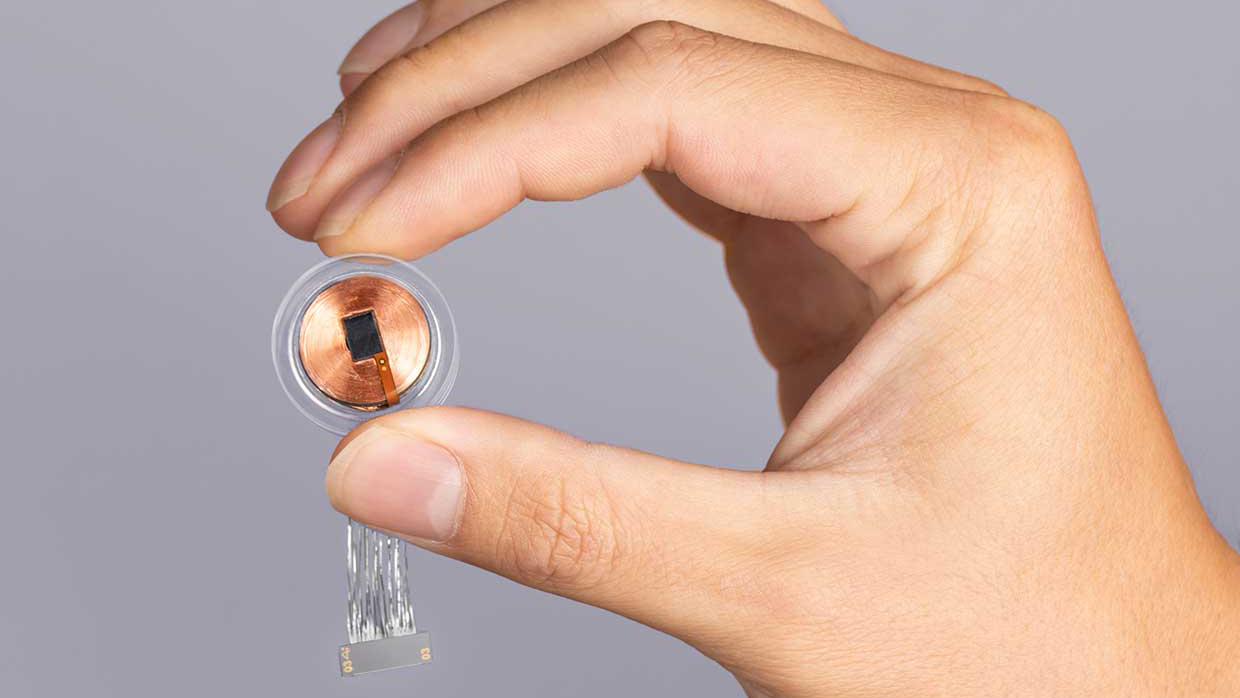
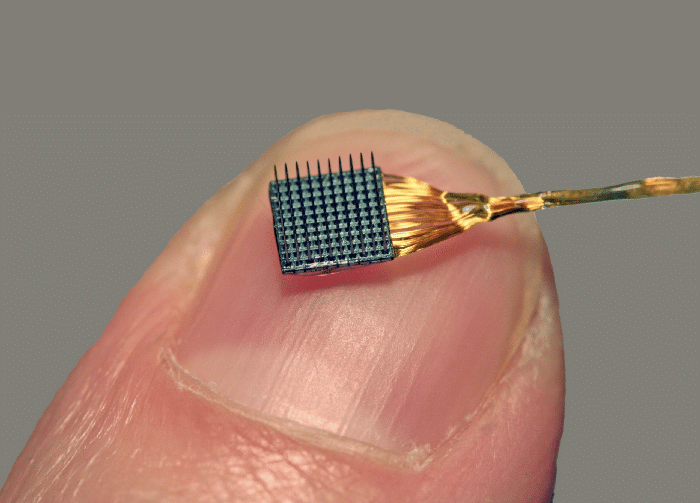
Past developments
As one might expect, the development of BMIs is intimately linked with progress in the larger field of neuroscience. It was not always obvious that the brain might be this big electrical machine, so any direct means of interfacing was impossible without some comprehension of the basic mechanisms involved. Thankfully, by the late 1700s the Italian scientist Luigi Galvani revealed the electrical nature of neurophysiology and opened the way to generations of neurophysiologists who have improved our understanding of the nervous system and developed various electrode technologies. To start recording the brain, it was necessary to understand that there were underlying signals that might contain useful information.
Once this was understood, as illustrated in (Hong & Lieber, 2019), the number of simultaneously recorded neurons by implantable electrodes approximately doubled every 7 years since the 1970s. This pursuit of scalability in neural recordings came from the (fairly reasonable) belief that acquiring more neuron signals in a robust manor would increase BMI usability and performance provided the decoding algorithms keep up. Of course, many challenges stood (and stand) in the way of ever-increasing neuron tracking. According to the authors, this relatively (when compared to Moore’s law) slow exponential increase is due to three factors that make the scaling difficult. The first is that increasing electrode count without inducing extra tissue damage is difficult, the second is that designing lightweight I/O interfaces capable of handling thousands of electrodes remains a challenge and finally, scaling the electrodes themselves isn’t straightforward as electrode impedance and thermal noise need to be kept within reasonable boundaries. The combination of these challenges explains the slow rise of the number of neurons recorded by electrophysiological recordings at the single-neuron level and gives a good overview of the direction of past research efforts. If each of these technical challenges is further addressed and if past trends are to be believed and used for extrapolation (a skeptical empiricist might argue quite the contrary - yes, I’m in the middle of reading Taleb’s Black Swan) then future discoveries will slowly but surely increase the number of recorded neurons by chipping away at these difficulties. So, what does all of this look like - concretely - in terms of recording devices ? The actual recording technologies (hardware) used to perform these recordings has evolved from Tungsten microwire electrodes, to Silicon probes to Tetrodes, each step addressing the concerns raised above.
Current State of the Art
Going back to non-invasive BMIs, a few prominent examples include Facebook’s Reality Labs (former CTRL-Labs) wristband or the Kernel headmount. Although at the time of writing it doesn’t seem Facebook has done much with their technology, it supposedly relies on capturing the electrical signals directly from the wrist to control an external device. Aside from the obvious non-invasive advantages, this will always be limited in the scope of possible applications. Signals travelling down to the wrist are already fairly specific, using them can make for some neat demos but doesn’t perhaps qualify as inspirational science. The headmount created by kernel, which I discovered on Lex Fridman’s podcast, seems to hold a little more promise when thinking about the non-invasive direction. According to the website, Kernel Flow is a non-invasive, full-coverage, optical headset that gives precise measures of brain activity. Crudely, it throws photons at your brain and analyzes how they scatter to infer what is going on inside. As it has not been tested clinically, we need to be cautiously optimistic about what this kind of tech might bring as it is still very much in the realm of experimental science.
The SoA in invasive BMIs is changing rapidly and quite exciting. I have personally worked with some ultrasoft mesh nanoelectronics which have a range of properties making them good candidates for the next generation of invasive technologies. This is in the same pursuit of scalability and durability that has marked the historical developments of BMIs. Be sure to go over to the publications page to check that out ;). There is also Neuralink’s system which can supposedly track thousands of neurons and uses soft, precisely placed flexible threads. Given the number of neurons in the brain (~100 billion) this still seems to fall short of what we would need to gain system-wide understanding and tracking but is nonetheless exciting.
Ultimately, although we have already been able to achieve remarkable milestones, (Anumanchipalli et al., 2019), (Willett et al., 2021)) in BMI development thanks to fixed arrays, the future seems to lie within these softer, denser technologies which will need to be accompanied by more scalable decoding algorithms.
References
-
Novel electrode technologies for neural recordings
-
Speech synthesis from neural decoding of spoken sentencesNature 2019
-
High-performance brain-to-text communication via handwritingNature 2021